Jun Huang
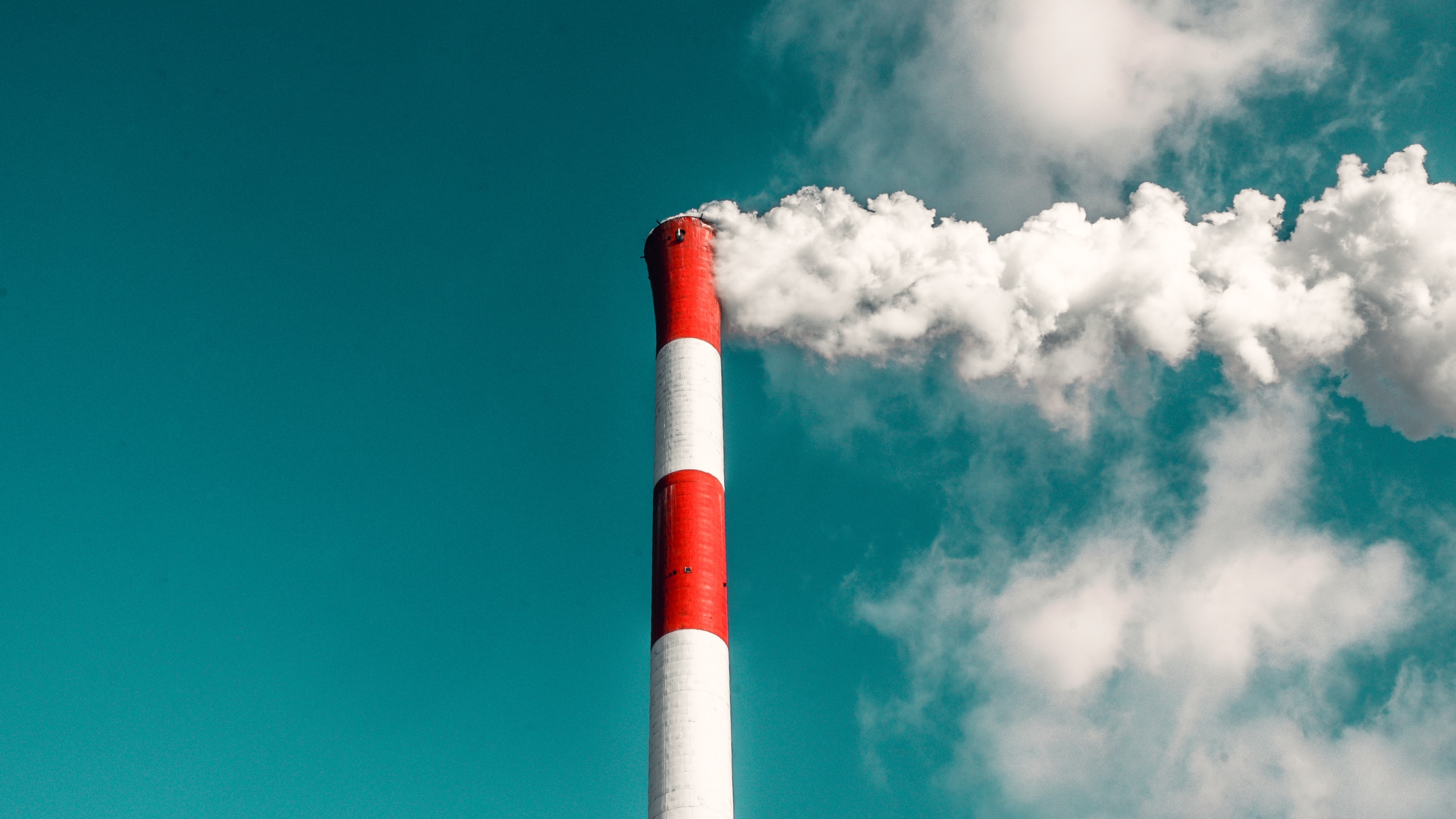
Turning greenhouse gas into clean fuel
What if it were possible to productively use two of the greenhouse gasses currently pitching the planet into environmental catastrophe, to produce a fuel that can power heavy machinery where the only by-product can be used as food for farm animals?
The ‘secret’ ingredient that makes this possible is a super-stable catalyst that, when combined with CO2 and the natural gas methane, produces hydrogen. The research team in my Catalysis Engineering lab at the University of Sydney has developed a super-stable catalyst for the CO2 reforming of methane (dry reforming) to produce hydrogen.
We believe this is a promising step in the quest to tackle excessive greenhouse gas output and ultimately sustainability. In other words, a world where the process of production does not threaten our environment but rather seeks to return it to a natural balance.
Now that I have your attention – how it works
Currently, fossil fuels are widely used by steam methane reforming or coal gasification to produce hydrogen. However, we proposed dry reforming of methane: steam reforming uses water instead of carbon dioxide as one of the feedstocks, which cannot reduce the CO2 emission and also consume huge amount of freshwater.
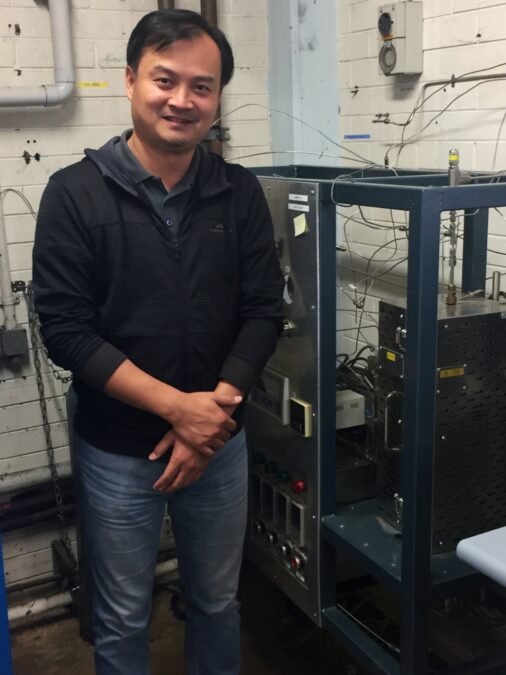
For example, if hydrogen production (currently 60 megatonnes per year) was from dry-reforming instead of steam reforming, nearly 0.5 gigatonnes per year of CO2 would be removed immediately, matching the target set for 2030 in the global sustainability roadmap for decarbonisation. Currently, the commercialisation of this process is impossible due to the lack of stable dry-reforming catalysts.
Now my group has developed new catalysts using Australian nickel feedstock, which is super-stable over 1,200 hours in dry-reforming (patent in process: CDIP 2020-143; FPA: M53127426). It is a new world record, more stable and easier to manufacture than the last record of 800 hours. It will also be significantly cheaper.
We also developed the pilot plant for the potential industrial application of dry reforming process for combining CO2 reduction together with sustainable hydrogen production.
Hydrogen and its uses
The market demand for hydrogen is huge. We envisage the final users of this totally clean hydrogen would include chemical and energy industries.
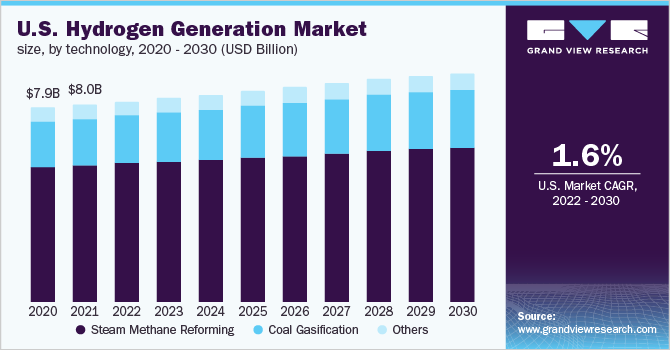
Hydrogen is the preferred fuel in Australia’s sustainable target and reducing greenhouse gases is another target. The development of catalysts and catalytic process in this proposal aims to provide hydrogen for multiple industries and applications such as fuel in fuel-cells, as a feedstock in industrial oil refining, as a raw material for ammonia production. It can be further converted as diesel substitution, methanol or dimethyl ether.
The energy demand produces the most CO2 through fuels for mobility and electricity, and raw materials for chemical industry.
Drying reforming can produce syngas on the scale of 20 to 30 gigatonnes per year to provide building blocks for the chemical industry, hydrogen gas for fuel cells, and fuels for power plants and existing vehicles, ultimately creating a large CO2 emission relief.
Water is the winner
Hydrogen is a clean fuel; it burns to water rather than a greenhouse gas. This should work well for industries with The Carbon Emissions Reduction Taskforce (CERT), creating a financial instrument tradable under a greenhouse gas Emissions Trading Scheme (ETS). In addition, for arid countries, this scenario relieves the water stress of steam reforming (the alternative process) for hydrogen production.
Australia is not rich in fresh water and freshwater shortage is a global challenge. Due to the lack of clean and drinkable water, steam reforming can hardly meet the goal of achieving long-term sustainability. Additionally, theoretically, if hydrogen production (~60 megatonnes per year) was from dry reforming instead of steam reforming globally, each year starting from now can remove ~ 0.5 gigatonnes CO2. This would be a tremendous number under the greenhouse gas Emissions Trading Scheme.
This could be an economical way to start a ‘net-zero emission energy systems’, remove vast quantities of greenhouse gases and produce useful chemicals and fuels simultaneously.
Image: veeterzy
Jun is Professor in the School of Chemical and Biomolecular Engineering and Director of the Laboratory for Catalysis Engineering at the University of Sydney. His research focuses on catalysis engineering and the scientific challenges involved in the field. He has been awarded an ARC Future Fellowship to further his research into the production of high-value chemicals from renewable alternatives.
Share
We believe in open and honest access to knowledge. We use a Creative Commons Attribution NoDerivatives licence for our articles and podcasts, so you can republish them for free, online or in print.